Introduction
Currently, petroleum fossil fuel is society’s principal source of liquid fuel and feedstock for the petrochemical industry. Requiring millions of years to form, petroleum is a finite resource on the human timescale. Moreover we are now aware of climate changes and the potentially severe repercussions thereof that result from greenhouse gas emissions, including carbon dioxide release from fossil fuel combustion. With sustainability and environmental impact at the forefront of global concerns, initiatives to develop biofuels and bio-based chemicals have garnered great interest and increasing investment from public and private sectors.
Biomass is renewable, carbon-neutral, and can be processed into a number of different products. Corn-based bioethanol from the USA and sugarcane-based bioethanol from Brazil account for the majority of global biofuel supply. Global biofuel production was estimated at 1.8 million barrels per day in 2011 and is forecasted to rise to 4.0-5.9 million barrels per day by 2040 [i]. However US biofuel production is expected to remain flat at 1 million barrels per day from 2011 to 2040 where decreasing use of bioethanol/gasoline blends will be offset by the market entry of drop-in biofuels [ii].
A major concern regarding such food crop-derived biofuels is the conflict with food supply resulting from diverting prime agricultural land towards fuel production. Second generation lignocellulosic biofuels avoid this conflict by utilizing cellulose in non-food parts of plants (such as corn stover) to produce ethanol. The major disadvantage of lignocellulosic biofuels is that they are more expensive to produce – current processes fall short of price parity with fossil fuels, government subsidies notwithstanding. One of the key additional costs is the pretreatment required to release cellulose from heteropolymeric lignocellulosic fibres containing lignin, cellulose, and hemicellulose; where lignin is the major obstruction.
Ironically, lignin valorization is conceptually powerful as a complement to cellulosic fuel production. Lignin-based high-value products could drive petroleum-competitive cellulosic fuels. Lignin is a major component of lignocellulosic biomass; it contributes 17-21% of the weight of corn stover for example[iii], and can form up to 35% of the weight of woody biomass. The major difficulty of lignin valorization is its recalcitrance to processing: its chemical and structural heterogeneity, and its insolubility in water impede handling and further chemistry. By consequence, the vast majority of lignin is considered “waste” and is combusted for low-value heat and power in biorefineries and pulping mills.
Nevertheless, interest in lignin is strong and getting stronger. The International Lignin Institute dates from 1991 [iv]. National lignin-focussed networks have sprung up recently [v], as well as a biennial international conference on lignin biochemicals. The technology to process biomass into renewable chemicals, especially aromatics such as benzene, toluene, and xylene (BTX) has begun pilot scale trials. Energetically, it is significantly more efficient to depolymerise and reduce existing aromatic structures than it is to build them from linear hydrocarbons. This development is important as an opportunity to pursue multiproduct biorefinery models where high-value bio-based chemicals are co-produced alongside low-value biofuels. Moreover the abundance of U.S. shale gas that was recently publicized can be expected to drive non-energy lignin uses by providing cheap heat and power. The ideal scenario is to derive chemicals from lignin, thus upgrading a waste stream to a major source of revenue.
Ionic Liquids for biomass treatment and co-valorisation
Ionic liquids have generated great enthusiasm with regards to biomass pretreatment by virtue of being very effective at dissolving crystalline cellulose and otherwise recalcitrant lignin, then allowing selective precipitation of high-purity biomass fractions. Moreover the liquid phase of ionic liquid-solubilised lignin is amenable to further chemistry such as is not otherwise possible with native lignin. The major caveat is that ionic liquids are currently very expensive and need to be recovered for high efficiency recycling.
Through use of this ionic liquid platform, lignin can be processed into a number of useful products. Lignocellulosic biomass or another source of polymeric lignin is dissolved in ionic liquid [e.g. C2mim-OAc]. The desired fraction, here lignin, is selectively precipitated and separated from the supernatant, or alternatively isolated by liquid-liquid extraction. Depending on the process conditions used, the lignin fraction may be partially or completely depolymerised; partially or completely derivatised; or extracted, and depolymerised and/or derivatised by additional chemistry.
This process can produce low molecular weight and monomeric non-polar aromatic compounds. Principal monomeric products include vanillins, guaiacols, and eugenols. These products can then be processed by reductive chemistry into benzene, toluene, xylene (BTX), and phenol. Alternatively or in parallel (with different yield efficiencies), another process can yield low molecular weight and monomeric aldehydes and aromatic aldehydes. These aldehyde compounds can be converted into amines and thereon to ammonium salts, thereby forming various ionic liquids.
Ionic liquid treatment of lignocellulosic biomass therefore supports producing the following products of interest: BTX, phenol, vanillin, and a new ionic liquids. Importantly, some of the ionic liquid products are suitable for efficient lignin solubilisation. This allows for a closed loop process in which biomass-derived ionic liquids can be used in the treatment of additional biomass. As the cost of ionic liquids is the major barrier to their uptake, the manufacture of ionic liquids from cheap biomass or other polymeric lignin presents a well-positioned value proposition that is strengthened by the ability to feedback ionic liquid product into the process input, thereby reducing a major source of process cost.
Work at JBEI funded by the DoE has demonstrated lignin-based ionic liquids from model compounds that simulate biomass lignin. The next step would be to demonstrate lignin-based ionic liquids from natural biomass and/or from commercially available technical lignin (e.g. Kraft lignin). JBEI has demonstrated lignin-based aromatic chemicals from raw biomass and technical lignins. There, catalytic and other reaction conditions, particularly based on feedstock variation, need to be optimised. Both processes are on the milliliter scale and need scaling analysis for up to 5-10 thousand liters.
Market overview
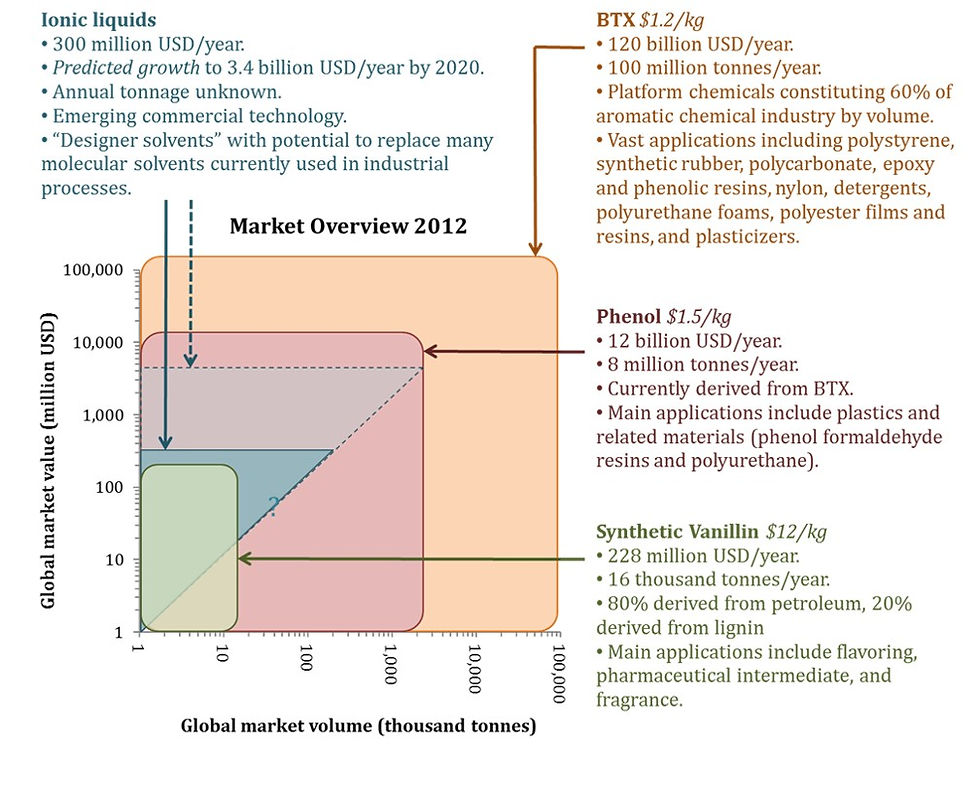
Market for BTX and phenol
The petrochemical industry dominates BTX and phenol production. Most BTX is fractionated from the catalytic reformate of naphtha. In turn, most phenol is made from BTX via cumene. These platform chemicals, particularly BTX, are the backbone of the aromatic petrochemical industry, of which BTX alone constitutes 60% by volume. The applications of BTX and phenol are vast. Some examples are given in Figure 2.
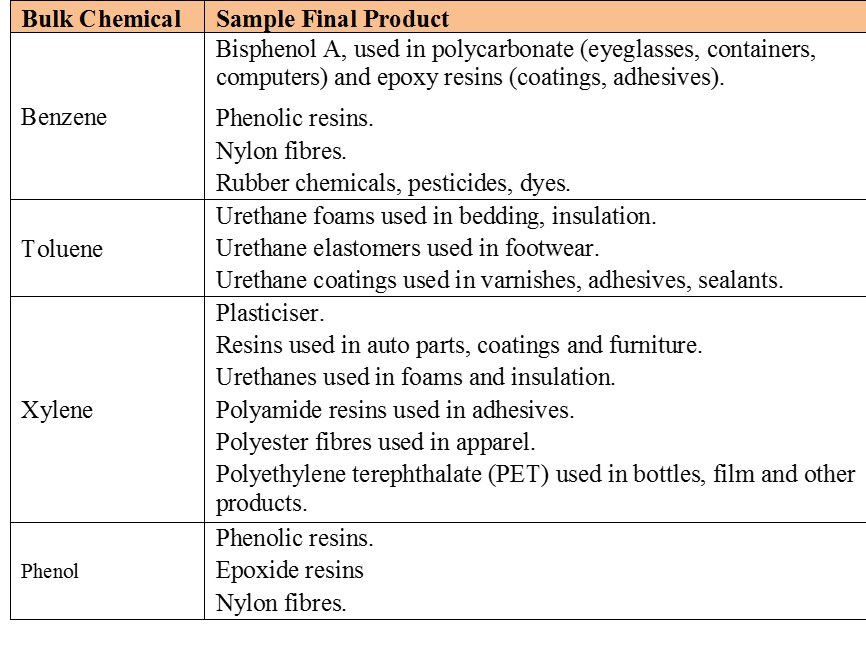
The global phenol market value is estimated at 12 billion USD and is forecasted to grow at a 3.9 % CAGR from 2013 through to 2020. 8 million tonnes of phenol were produced in 2010. An order of magnitude larger, the global BTX market value is estimated at 120 billion USD and is forecasted to grow at a 4.4 % CAGR from 2013 through to 2020. In 2010 BTX was produced in the following volumes in million tonnes per year: benzene - 40.2, toluene – 19.8, and xylene – 42.5 .
Such large and established markets present a low risk of insufficient product uptake, particularly because public sensibility to environmental issues has grown a very large demand for “green” consumer products. For example large companies such as Coca Cola are marketing themselves as environmentally conscious by purchasing carbon credits to offset carbon costs of product manufacture and through recycling schemes . The offer of sustainable, carbon-neutral plastics should be met with enthusiasm.
Moreover as platform chemicals, “green” BTX and phenol can take advantage of existing petrochemical infrastructure for processing and distribution. These products therefore target a large, stable, and easily accessed market. However even with a “green” premium on pricing, a high volume output will be necessary to drive profitability through economies of scale.
Market for Synthetic Vanillin
Vanillin bean-derived vanillin accounts for less than 1% of global supply (but 16% of global market value). Although lignin has been a feedstock for synthetic vanillin since 1937 the process, which sourced lignin from spent sulphite liquor, raised environmental issues and fell into disfavour. Currently only one major manufacturer of lignin-based vanillin remains: Norwegian company Borregaard, who claims a 20% global market share. Synthetic vanillin produced from crude oil-derived guaiacol accounts for the remaining 80% .
Vanillin is an end product whose principal uses are as a flavouring (82 %), a pharmaceutical intermediate (13 %), and a fragrance (5 %). The global synthetic vanillin market is estimated at 228 million USD and is forecasted to grow at a CAGR of 2% in Europe and the USA, although the annual growth rate is expected to hit 10% in China. 16 thousand tonnes of synthetic vanillin were produced in 2010.
Although vanillin alone is too small a market to be regarded as strategic, it is an established, high value product representing an opportunity for early revenue. This may be particularly important as BTX and phenol production is being ramped up to hit economies of scale, and ionic liquids are still penetrating the industrial solvent market.
Market for Ionic Liquids
Although in the mid-1990s ionic liquids were esoteric curiosities to a narrow field of electrochemists, the field is now growing rapidly. Already in 2011 there were 150 companies involved in the science and markets of ionic liquids. This number is expected to rise to several thousand by 2030. Several companies now have multi-ton capabilities for ionic liquids supply.
Ionic liquids represent the basis for a new industrial technology. With considerable ease the cation or anion or both can be changed to optimize the relative solubilities of reactants and products, the reaction kinetics, the liquid range of the solvent, the cost of the solvent, the intrinsic catalytic behaviour of the media, and the air-stability of the system. Unlike the current use of molecular solvents, ionic liquids can be designed to optimize a reaction (with control over both yield and selectivity) rather than let the solvent dictate the course of the reaction .
Additionally, ionic liquids are perceived as “green chemistry”. Ionic liquids reduce the need for more hazardous substances. They are not flammable and release no significant fumes to the environment (they have extremely low volatility). The adoption of ionic liquids as industrial solvents is expected to increase workplace safety and reduce the generation of hazardous waste. The major barrier to uptake is the cost of ionic liquids.
The global ionic liquid market is estimated at 300 million USD, but is predicted to reach 3.4 billion USD by 2020 . The USA and Germany lead the market (and R&D) with a 70% share, followed by China and Japan. Currently 300 ionic liquids are commercialized, compared to the 600 molecular solvents that find widespread use in industry. A predicted 1 million simple ionic liquids can be formed from combinations of known ions and anions, allowing up to 1 trillion possible ternary ionic liquid systems.
Although chemical engineering tends to be a conservative field, the penetration of ionic liquid technology has visibly begun and can only be expected to accelerate. Major players have already begun strategic movements, however the novelty and breadth of ionic liquid technology means this market is fertile for product innovation and significant growth opportunities by smaller companies.
Feedstock Supply
A stable and diversified supply chain for lignin feedstock must be identified to justify commercial implementation of lignin-dependent technology. There are four major sources of lignin: (1) lignin waste from the Pulp & Paper industry, (2) lignin waste from the Lignocellulosic Biofuels industry, (3) commercial lignin, and (4) raw biomass waste.
(1) Lignin waste from the Pulp & Paper industry
The Pulp & Paper industry produces lignin-rich pulping liquor as a waste product. Pulping liquor is typically combusted for heat and steam power, as well as to recover spent chemicals. An estimated 45 million dry tonnes of pulping liquor are produced every year in the USA alone, of which 80% and 20% are generated through Kraft and sulphite pulping respectively .
Kraft pulping produces fibers of superior strength to sulphite pulping and black liquor as a waste product. Kraft lignin precipitated from black liquor is a highly refractory, water-insoluble material; for which reason it is considered intractable for upgrading to higher-value products. By contrast sulphite liquor contains water-soluble sulfonated lignin, which accounts for the majority of commercial lignin.
Pulping mills are highly energetically integrated facilities, yet modern mills operate on an energy surplus. Debottlenecking of thermal load-limited pulp production is possible by precipitating Kraft lignin from black liquor, thus reducing its energy content. This Kraft lignin is often discussed of as solid fuel for other areas of the pulp mill.
(2) Lignin waste from the Lignocellulosic Biofuels industry
The Lignocellulosic Biofuel industry is gathering momentum: at least 600 million litres in additional production capacity has or will come online in the period 2012- 2014 (see Figure 3). In most cases, the lignin output of the biorefinery is combusted for heat and power.
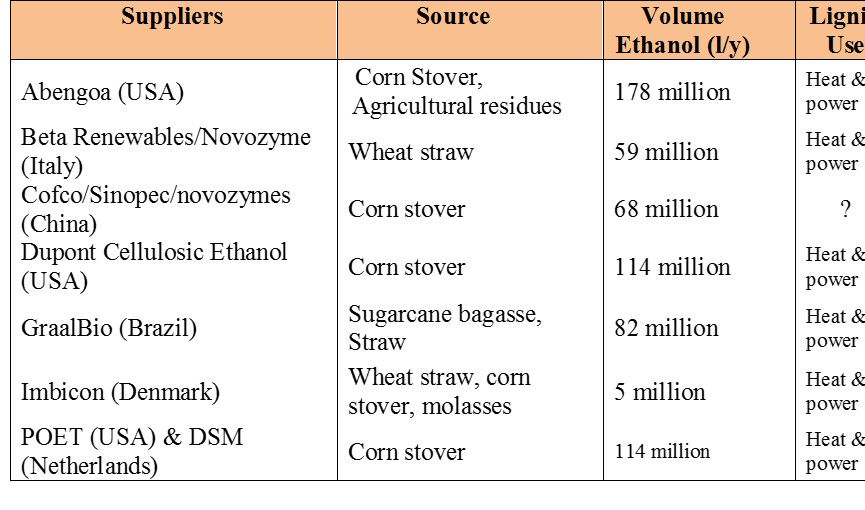
Similarly to the Pulp & Paper industry, pricing of lignin feedstock above its energy content should sufficiently incentivize biorefineries to accommodate lignin offtake. As lignin valorization is a prominent concept in scientific and industry literature, especially in relation to biorefinery economics, I would expect biorefineries to be primed for this collaboration.
Unlike the pulping process, commercial lignocellulosic ethanol is a nascent technology. The large variability in industrial processes used lead to lignin waste streams of differing physical and chemical properties. Technology capable of processing a diversity of lignin types will be advantaged with wider feedstock access.
(3) Commercial lignin
The valorization of lignin is already pursued on the commercial scale, almost entirely as low-value lignosulfonates (see Figure 4). These find use mainly as emulsifiers, dispersants, and binding agents. Although likely far more expensive than the use of waste lignin as feedstock, the use of commercial lignin offers some advantages. Such companies are demonstrably willing to supply lignin; have established distribution channels; and may have the capability to tune and/or make more uniform the physicochemical properties of their product, which may be advantageous for downstream process optimization.

(4) Raw biomass waste
A significant amount of raw biomass is discarded annually, essentially because its value does not cover handling and transport costs. Such biomass includes forestry waste such as logging residues, trimmings and cuttings from merchantable trees, small tress culled against fire risk; agricultural waste such as crop residues; and urban wood wastes such as discarded furniture and wood used in the construction, remodeling, or demolition of buildings.
The following figures are estimates of the amount of lignocellulosic biomass annually available in the USA from these sources that are also suitable for input into bioenergy and bio-based product applications :
• Forestry waste: 88 million dry tonnes per year.
• Agricultural waste: 147 million dry tonnes per year.
• Urban waste: 32 million dry tonnes per year.
In using these feedstocks one could compete with cellulosic biofuel companies, especially with regards to agricultural wastes such as corn stover, although the higher value of bio-based chemicals versus bio-based fuels may lend competitive advantage. However the use of raw biomass as feedstock would require additional pre-treatment as well as the handling of cellulose and hemicellulose fractions.
Conclusions
The technology presented in this artcile addresses two new markets: (i) “green” aromatic chemicals (Chemicals industry) and (ii) ionic liquids (Materials industry), whilst also being able to secure early revenue through vanillin. Lignin-based BTX and phenol are an ideal value proposition for industries striving to reduce their environmental footprint, which most industries are. Moreover the market is well established, diversified, and very large. Lignin-based ionic liquids offer new solvent technology with less pollution and better workplace safety, produced from cheap feedstock. The market is growing very rapidly. All these lignin-based products have significantly more value than lignin feedstock combusted for heat and power.
However separate bio-based fuel and bio-based specialty chemical production processes do not benefit from any obvious synergies other than sharing feedstock, and the important opportunity to reduce ionic liquid process costs by in-house manufacture and usage. The feasibility of these two processes need to be evaluated, including the final stages of the technical feasibility (e.g. validating the raw biomass/technical lignin feedstock for ionic liquid products), as well as economic feasibility.
To this end, scaling analysis is crucial to explore the sensitivity of yields and other important parameters to reaction conditions. Once process yields and other specifications are determined at large scale, detailed technoeconomic analysis will be necessary. This will provide sufficient evidence of the feasibility to commercially upgrade partially-processed lignin waste to valuable chemicals.
[i] US EIA Annual Energy Outlook April 2013 page 59.
[ii] US EIA Annual Energy Outlook April 2013 page 80.
[iii] Karin Ohgren et al. (2007) “Effect of Hemicellulose and Lignin Removal on Enzymatic Hydrolysis of Steam Pretreated Corn Stover” 98 Bioresource Technology 2503.
[iv] Website: www.ili-lignin.com, accessed 19th August 2013.
[v] Website: www.ligninplatform.wur.nl, accessed 19th August 2013.
Kommentare